- 1School of Materials and Energy, Guangdong University of Technology, Guangzhou, China
- 2School of Materials Science and Engineering, Nanyang Technological University, Singapore, Singapore
- 3Key Laboratory of Materials Processing and Mold (Zhengzhou University), Ministry of Education, Zhengzhou University, Zhengzhou, China
- 4Hefei National Laboratory for Physical Sciences at the Microscale, Department of Materials Science and Engineering, Key Laboratory of Materials for Energy Conversion, Chinese Academy of Sciences (CAS), University of Science and Technology of China, Hefei, China
- 5Dalian National Laboratory for Clean Energy (DNL), Chinese Academy of Sciences, Dalian, China
- 6State Key Laboratory of Fire Science, University of Science and Technology of China, Hefei, China
Na-ion batteries (SIBs) are anticipated to capture a broad development space in the field of large-scale energy storage due to the abundant sodium resources. High-performance cathode materials are very critical. VOPO4⋅2H2O with a two-dimensional (2D) layered structure is a very promising candidate for SIBs because of its high working voltage and theoretical specific capacity. Herein, a simple one-step reflux method is designed to fabricate a cathode of VOPO4⋅2H2O nanosheets. It exhibits a high average operating potential of ∼3.5 V, remarkable specific capacity (e.g., 135 mAh g–1 at 0.05 C), favorable high current charge-discharge ability (e.g., 58 mAh g–1 even at 20 C) as well as extralong cyclability (e.g., 0.026% capacity fading rate for per cycle at 20 C during 1000 cycles). The kinetic analysis implies that the superior sodium storage performance is mainly benefiting from the advantages of unique nanosheet structure, accelerating the rapid Na-ion diffusion.
Introduction
The dramatic growing of the portable electronic technology has stimulated the fast development of rechargeable batteries owing to high safety, low cost, availably, scalability and environmental benignity (Deng et al., 2020; Tang et al., 2020; Xie et al., 2020). As a pioneer, Li-ion batteries (LIBs) have become a leader in commercialization since their high energy densities and stable cycling performance (Tan et al., 2018; Yang et al., 2019, 2020). However, the issues of limited reserves, uneven distribution and high price of lithium resources perplexed their large-scale energy storage applications in future (Wang et al., 2017; Guo et al., 2020) Among the post LIBs, Na-ion batteries (SIBs) have caused wide increasing concern due to the cheap price, rich reserve and even distribution of sodium resources in the crust of the earth (Chen S. et al., 2017; Yu et al., 2019; Zhao et al., 2019). However, the bulkier radius [1.02 (Na+) vs. 0.76 Å (Li+)] makes the Na-ion diffusion more sluggish than that of Li-ions, resulting in the larger volume change, the lower special capacity and the poorer cyclical stability, which limit the further development of SIBs in large-scale energy storage system (Wang et al., 2018; Liu et al., 2020). Therefore, designing/exploring novel advanced electrodes for SIBs to facilitate fast Na-ion transfer is the top priority at present (Li C. et al., 2020; Wang B. et al., 2020).
Recently, two-dimensional (2D) layer electrodes have shown the promising electrochemical property due to their unique 2D ion transport corridor, short ion diffusion distance and adjustable interlayer space, which are beneficial to promote the Na-ion insertion/extraction reactions (Huang et al., 2014; Shi et al., 2019; Verma et al., 2019). Among them, 2D hydrated vanadium oxyphosphate (VOPO4⋅2H2O) has received considerable attention as the promising cathode materials for SIBs because of its high redox reaction potential (∼3.5 V), high theoretical specific capacity (166 mAh g–1), and various valences reactions (e.g., V5 +∼V3+) (Chen N. et al., 2017; Wang J. et al., 2020; Xiong et al., 2020). For example, the bulk VOPO4⋅2H2O synthetized by Peng et al. (2017) can release a delightful reversible specific capacity of 151 mAh g–1 at the testing rate of 0.1 C. It however displays inferior rate capability (e.g., ∼50 mAh g–1 at a high electrical current density of 20 C) and cycling performance (only 51% capacity retention over 500 cycles at 5 C). Another bulk VOPO4⋅2H2O cathode prepared by Zhu’s group, which although delivers an acceptable discharge capacity (∼140 mAh g–1 at 0.1 C), it shows a poor high current charge-discharge ability with almost no capacity at 5 C (Zhu et al., 2016). This is mainly because of the large bulk morphology, which prolongs the diffusion distance of sodium ions. Thus, it is highly desirable to build nanostructured VOPO4⋅2H2O to enhance the sodium storage kinetics.
In this work, VOPO4⋅2H2O nanosheets (thickness: 20–40 nm) are developed and utilized as the cathode material to enable fast and reversible sodium ion intercalation/deintercalation, so that it exhibited high reversible sodium storage capacity (135 mAh g–1 at 0.05 C), remarkably improved rate capability (58 mAh g–1 even at 20 C) and extralong cycle stability (e.g., capacity retention of 74% at a high rate of 20 C after the 1000th cycle). In addition, the VOPO4⋅2H2O nanosheets also showed rapid Na-ion diffusivities exceeding 1.7 × 10–13 cm2 s–1 due to the convenient Na+ transport channel provided by the nanosheets structure. The outstanding SIB performance of the VOPO4⋅2H2O nanosheet cathode makes it to be a very popular candidate with increasingly wide utilization in large-scale energy storage system.
Materials and Methods
Synthesis of VOPO4⋅2H2O Nanosheets
All reagents were purchased from Sinopharm Chemical Reagent Co., Ltd. (China) and used directly without any treatment. The VOPO4⋅2H2O nanosheets was synthesized by a feasible reflux method. Specifically, 5.0 g of vanadium pentoxide powders (V2O5) and 120 mL of deionized water (DI H2O) were put into a conical flask and then 30 mL of the concentrated phosphoric acid (85% H3PO4) was added. The suspension was then refluxed at the heating temperature of 100°C for 18 h under vigorous stirring. After the reaction completed, it takes a little time to cool to room temperature, and the yellow-greenish sample was finally gathered by centrifugation, washing (water, ethanol and acetone), and vacuum drying (60°C for 5 h). For comparison, the bulk VOPO4⋅2H2O sample was synthesized by a traditional ball milling approach. 5.0 g of V2O5, 120 mL of DI H2O, and 30 mL of 85% H3PO4were accurately weighed and added to the agate jar in proper order. After that, the milling equipment operated along a single orientation with the optimal rotate speed of 500 rmp min–1 for 18 h, and then it was centrifuged, washed and vacuum dried to yield bulk VOPO4⋅2H2O sample. Here, it is worth mentioning that the as-obtained samples were stored in a glove box filled with argon atmosphere.
Characterization
The X-ray diffraction (XRD) patterns were carried out on a Rigaku SmartLab polycrystall X-ray diffraction with Cu Kα X-ray source (λ = 1.54056 Å) scanned in the 2θ range of 10–60°. The Fourier transform infrared spectroscope (FTIR) was recorded on a UV-3600 Plus spectrometer. The thermal gravimetric analysis (TGA/DSC3+) were tested within the temperature range of 25–750°C in air atmosphere (heating rate: 10°C min−1). The morphology was studied by employing a Hitach SU-8220 field-emission scanning electron microscopy (FESEM) at 10 kV accelerating voltage and a FEI Tecnai G2f20s-twin 200 kV transmission electron microscopy (TEM) instrument.
Electrochemical Measurement
The electrochemical measurements of the as-obtained VOPO4⋅2H2O cathodes were investigated in 2032-type coin cells, which were assembled in an argon-filled glovebox equipment, where both H2O and O2 levels were less than 0.1 ppm. For the cathode electrode part, it was prepared by mixing VOPO4⋅2H2O, carbon nanotubes and polyvinylidene fluoride (the mass ratio = 6:3:1) in the N-methyl pyrrolidone solvent, and then the well stirred slurry was coated evenly on an aluminum foil and dried at 60°C in a vacuum oven for 12 h. Na metal was used as the counter electrode. Glass fiber membrane was acted as separator. And a solution of 1 mol L–1 NaClO4 in propylene carbonate (PC) and ethylene carbonate (EC) (1:1 v/v) with 5% fluoroethylene carbonate (FEC) was selected as electrolyte. The galvanostatic discharge-charge measurements were carried out on NEWARE system within the cutoff voltage window of 2.5–4.3 V versus Na+/Na. Electrochemical impedance spectroscopy (EIS) with the testing frequency range of 105 to 10–2 Hz and cyclic voltammograms (CV) at different scan rates were conducted by the Multi-Autolab M204 electrochemical workstation.
Results and Discussion
Figure 1A demonstrated the XRD pattern of the as-synthesized sample via applying a reflux method. All the presented diffraction peaks can be well indexed into the tetragonal VOPO4⋅2H2O (JCPDS files no. 84-0111, space group: P4/n) with no detected impurity peak, implying the as-synthesized sample is pure high-crystalline VOPO4⋅2H2O (Zhou et al., 2016). TGA analysis was employed to identify the water content of the as-obtained sample (inset in Figure 1A). It is showed a weight loss of ∼18% between 25 and 200°C, attributing to the evaporation of the crystal water and the content is determined to be two H2O molecules per unit formula of VOPO4, which is also in good agreement with the data from previous literatures (Zhou et al., 2018; Hyoung et al., 2019). The FTIR spectrum is shown in Figure 1B. The vibration peaks in the low wave number region (<800 cm–1) are represented the V–O stretching vibration. And the two intense broad bands at 1080 and 950 cm–1 are ascribed to P–O bond, implying the existence of [PO4] tetrahedral (Bao et al., 2011). Additionally, the band located at 1620 cm–1 is corresponding to the bending vibration of interlayer water molecules. And two characteristic bands at 3570 and 3400 cm–1 are relative to P–OH stretching vibration and OH stretching vibration of water molecule in VOPO4⋅2H2O, respectively (Zhou et al., 2014). Furthermore, the XPS analysis is also employed to confirm the surface compositions and oxidation state of vanadium in the VOPO4⋅2H2O nanosheets. As illustrated in Figure 1C, the P, O, and V elements are found in the XPS profile, consistent with the results obtained from the XRD test. It is worth mentioning that the C1s is used as the standard spectrum for calibrating system error, whose core-level XPS spectrum coupled with that of P 2p and O 1s are shown in Supplementary Figure S1. According to Figure 1D, the V 2p core-level shows the binding energies corresponding to V 2p2/3 peak and V 2p1/2 peak are 517.5 and 525.2 eV, respectively, confirming the main state of V5+ in the synthesized sample (Yang et al., 2018).
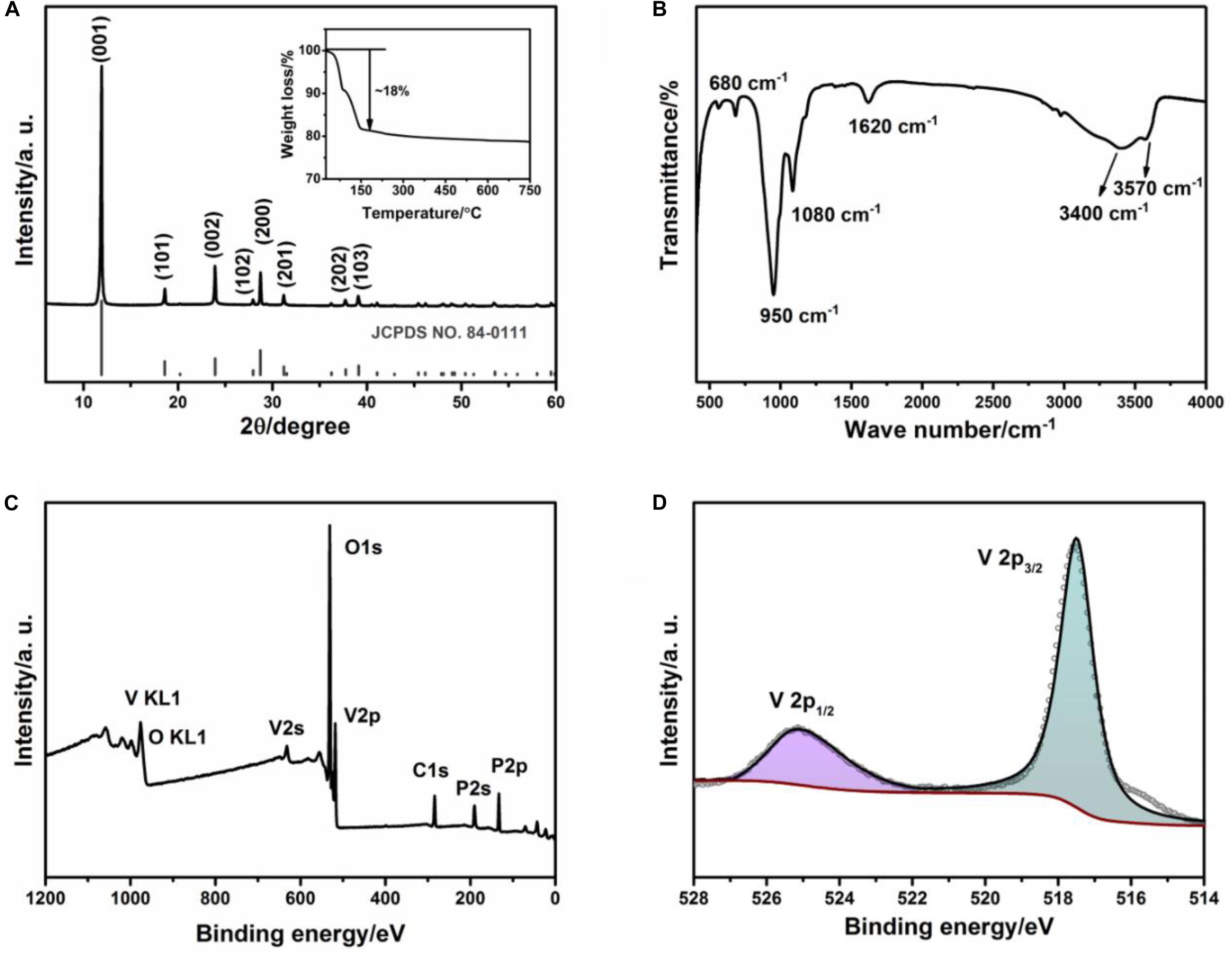
Figure 1. (A) XRD pattern and TG curve (inset); (B) FT-IR spectrogram; (C) XPS spectrum; (D) Core-level XPS spectrum of V2p of the sample prepared by a reflux method.
The morphology and detailed microstructure of the sample prepared by a reflux method were explored by field emission scanning electron microscopy (FESEM) and transmission electron microscopy (TEM). As presented in FESEM images in Figures 2a–c, the as-prepared sample shows numerous smooth-surfaced homogenous nanosheets with an approximately thickness of 20–40 nm integrated in an interconnected framework, which can stimulate fast Na-ions transportation and electrons diffusion. The TEM images (Figures 2d,e) further reveal the ultrathin feature of nanosheets as indicated by the almost transparent character of the nanosheets. The detailed crystalline structure of VOPO4⋅2H2O nanosheets was ulteriorly explored by high-resolution transmission electron microscope (HRTEM). As displayed in Figure 2f, the crystalline lattice distance of 0.351 and 0.319 nm is indexed to the (002) and (102) planes of the tetragonal VOPO4⋅2H2O, respectively.
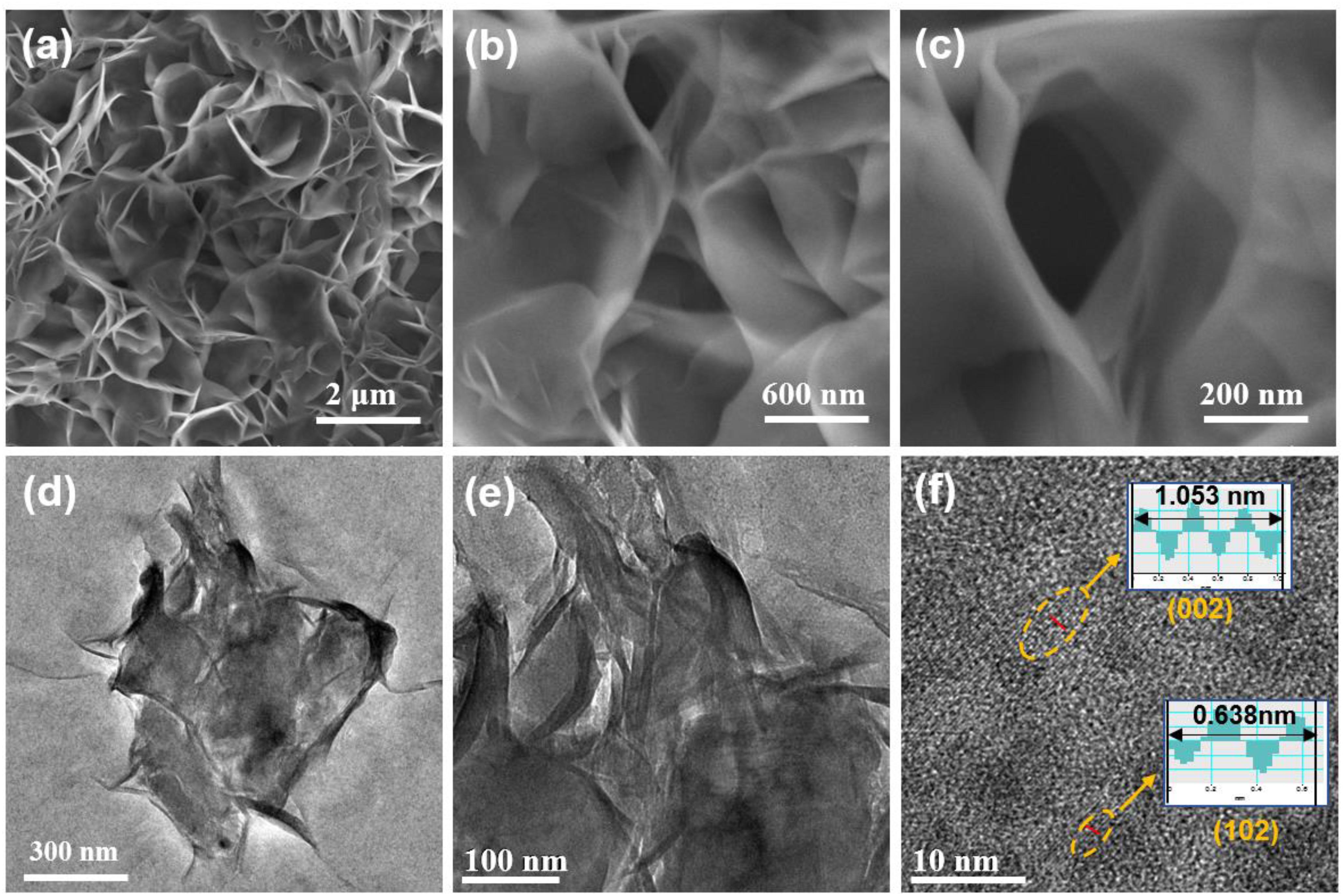
Figure 2. FESEM images of the VOPO4⋅2H2O nanosheets with different magnifications (a–c); TEM images of the VOPO4⋅2H2O nanosheets (d,e); HRTEM image of the VOPO4⋅2H2O nanosheets (f).
For comparison, the bulk VOPO4⋅2H2O sample was prepared by a ball milling method and its intrinsic basic characterizations are investigated and shown in Supplementary Figures S2–S6. It is evident that the SEM images of bulk VOPO4⋅2H2O control sample display an irregular large chunk morphology with the size of >2 μm, accompanied by obvious agglomeration (Supplementary Figure S6).
The electrochemical behavior of VOPO4⋅2H2O nanosheets and bulk VOPO4⋅2H2O as SIB cathodes were evaluated in a voltage window of 2.5–4.3V (vs. Na+/Na) by the galvanostatic charge and discharge test. As shown in Figure 3A, both samples exhibit the S-type charge/discharge profiles with an average working potential of ∼3.5 V (vs. Na+/Na), corresponding to the V4+/V5+ redox couple (Peng et al., 2017). The VOPO4⋅2H2O nanosheets display an specific discharge capacity of 135 mAh g–1 at a low current density of 0.05 C, which is remained at 123 mAh g–1 after 100 cycles with a 91% capacity retention (Figure 3B). During cycling, the Coulombic efficiency is always kept at ∼100%, suggesting a good reversibility. However, the specific discharge capacity of the bulk VOPO4⋅2H2O is only 109 mAh g–1 at 0.05 C, and a relatively low capacity retention ratio of 85% after the 100th cycle is illustrated. The inferior performance of the bulk material is mainly due to its large size, which makes the longer Na-ion diffusion path and some of the inner part of the active materials may not participate in the electrochemical reaction, resulting in the lower reversible specific capacity and quicker capacity decay. More importantly, owing to the unique nanosheet structure, the VOPO4⋅2H2O nanosheets exhibit a remarkably high rate capability. As displayed in Figure 3C, the specific discharge capacities of 127, 117, 111, 105, 99, and 93 mAh g–1 can be available at rates of 0.1, 0.2, 0.5, 1, 2, and 5 C, respectively. And even at high rates of 10 and 20 C, the reversible capacities actually reach 82 and 58 mAh g–1, respectively. And the discharge capacity can still be back to 119 mAh g–1 when the rate is returned to 0.05 C. In contrast, the rate capability of the bulk VOPO4⋅2H2O is much poor, which barely delivers 45 and 29 mAh g–1 at 5 and 10 C, respectively, and even almost no capacity at a high rate of 20 C. Meanwhile, Figure 3D shows the charge-discharge curves of VOPO4⋅2H2O nanosheets cathode at various current rates. It is observed that there is more and more obvious polarization phenomenon with increasing current rate, but the general S-type shape of the curves are still maintained, implying a favorable electrochemical stability of the VOPO4⋅2H2O nanosheets as cathode material for SIBs. Moreover, the long-term cyclability of VOPO4⋅2H2O nanosheets was also evaluated at 20 C (Figure 3E). Impressively, a reversible discharge specific capacity of 43 mAh g–1 can be maintained after 1000 cycles with a capacity retention of 74%. As examined by the ex situ XRD (Supplementary Figure S7) and SEM (Supplementary Figure S8), the slightly capacity decay is mainly because of the crystallinity deterioration and nanosheet morphology pulverization. Nevertheless, it is still much better than that of the bulk VOPO4⋅2H2O (i.e., 57% capacity retention over only 300 cycles at 20 C, Supplementary Figure S9).
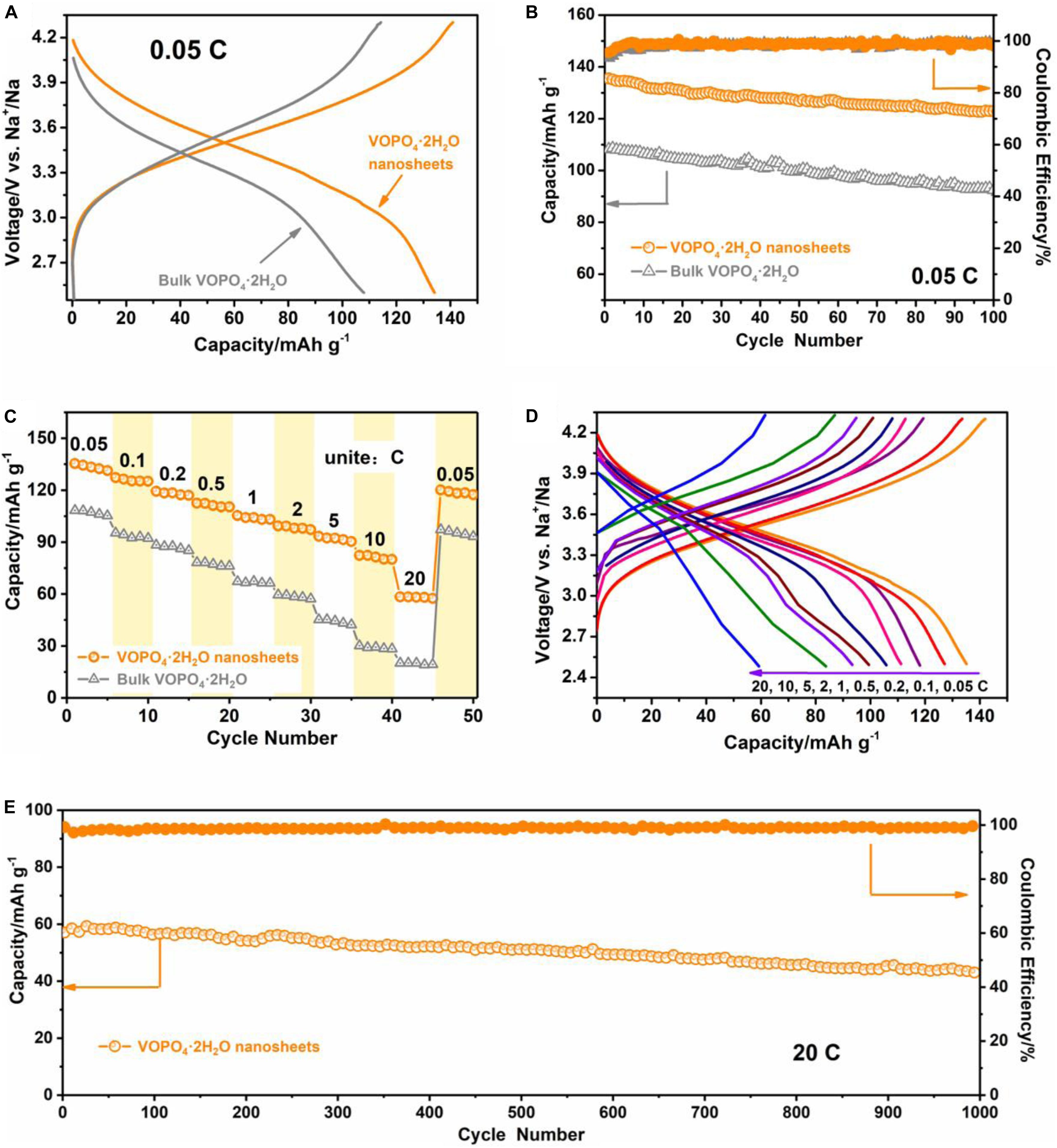
Figure 3. Sodium storage performance of the VOPO4⋅2H2O nanosheets and bulk VOPO4⋅2H2O. (A) the galvanostatic charge/discharge profiles at 0.05°C; (B) cycling performance at 0.05°C; (C) rate capabilities; (D) galvanostatic charge/discharge curves of VOPO4⋅2H2O nanosheets at various rates; and (E) long-term cycling behavior of VOPO4⋅2H2O nanosheets at 20°C (the cell was initially activated for five cycles at 0.05°C).
To further highlight the advantages of VOPO4⋅2H2O nanosheets, the electrochemical impedance spectrum measurement (EIS) and cyclic voltammetry (CV) are conducted to explore the charge transfer kinetics. The impedance spectra of the VOPO4⋅2H2O nanosheets together with the bulk VOPO4⋅2H2O sample were evaluated at the 5th fully discharged state. As displayed in Figure 4A, the Nyquist plots are comprised of one intercept-value at high frequency, one semicircle at intermediate frequency and one inclined line in low frequency area, which are consistent with the external circuit resistance (Rb), the charge transfer resistance (Rct), and the Warburg impedance (W), respectively (Guo et al., 2019). The Nyquist curves are fitted by the equivalent circuit as illustrated in Figure 4B, and the fitted impedance parameters indicated that the Rct-value of the VOPO4⋅2H2O nanosheets (211 Ω) is much smaller than that of bulk VOPO4⋅2H2O sample (332 Ω), implying the better electronic conductivity and faster charge transfer for the VOPO4⋅2H2O nanosheets. Figure 4C presented the CV curves of the VOPO4⋅2H2O nanosheets at diverse scan rate from 0.1 to 0.5 mV s–1. Obviously, the CV curves exhibited comparable shapes and gradually increased peak intensity along with the increase of scanning rates. And a pair of redox peaks is attributed to the V4+/V5+ redox couple reaction, being consistent with the previous literature (Zhu et al., 2016). In addition, the appearance diffusion coefficients of Na+ (DNa^+) in VOPO4⋅2H2O cathodes are also investigated on the basis of the CV technique and the Randles-Sevchik Equation (Li Q. et al., 2020):
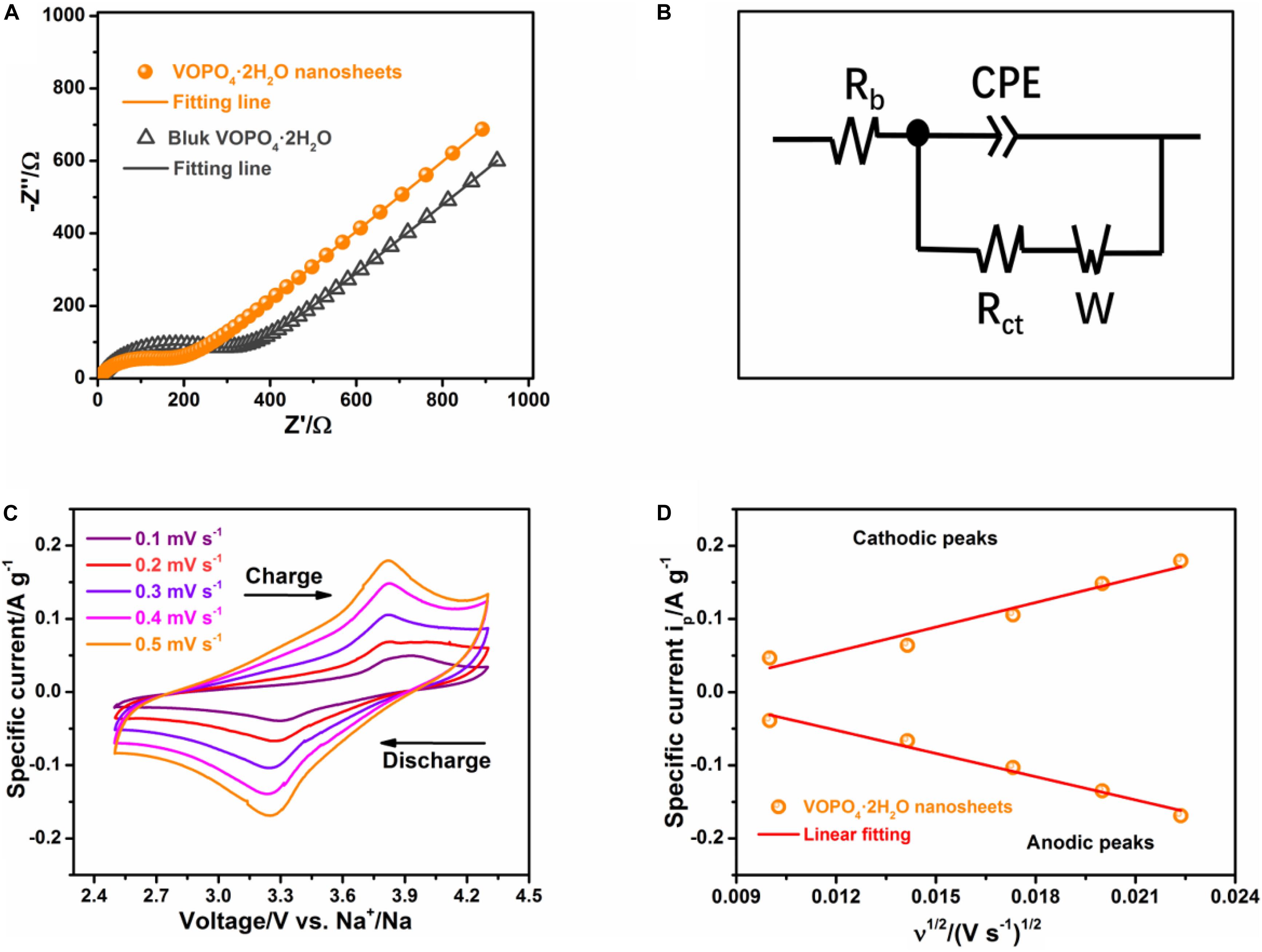
Figure 4. Electrochemically kinetic analysis of the VOPO4⋅2H2O nanosheets and bulk VOPO4⋅2H2O. (A) Nyquist plots; (B) equivalent circuit model of the impedance spectrum; (C) CV profiles of the VOPO4⋅2H2O nanosheets at 0.1–0.5 mV s–1, and (D) the linear relationship between the specific peak current and the square root of scan rate.
where ip is the specific peak current, n is the number of electron transferred, S is the active surface area of the cathode, the concentration of Na-ions in the VOPO4⋅2H2O is represented by CNa^+, and υ is scan rate. DNa^+ is determined by the slope of fitting linear of ip−υ1/2. As displayed in Figure 4D and Supplementary Figure S10, a linear relationship between the ip and the square root of the scan rate υ1/2 is found for both VOPO4⋅2H2O electrodes. Based on the slope value, the DNa^+ of VOPO4⋅2H2O nanosheets is determined to be ∼1.7 × 10–13 cm2 s–1, which is one magnitude larger than that of the bulk VOPO4⋅2H2O sample (∼1.9 × 10–14 cm2 s–1), indicating the rapid Na+ extraction/insertion reaction kinetics of the VOPO4⋅2H2O nanosheets.
Conclusion
In summary, a simple one-step reflux method was employed to synthetize the novel VOPO4⋅2H2O nanosheets (thickness: 20–40 nm), which can enhance the Na-ion diffusion kinetics. As a result, the VOPO4⋅2H2O nanosheets displayed a high average operating voltage (∼3.5 V), gratifying reversible capacity (e.g., 135 mAh g–1 at 0.05 C), remarkably improved rate capability (e.g., 58 mAh g–1 even at 20 C) and excellent cyclic stability (e.g., 74% capacity retention at 20 C over 1000 cycles). The outstanding electrochemical performance of the VOPO4⋅2H2O nanosheets makes it a promising cathode material in rechargeable SIBs.
Data Availability Statement
All datasets generated for this study are included in the article/Supplementary Material, further inquiries can be directed to the corresponding author/s.
Author Contributions
XR and YY directed the project. XZ, DY, WL, and YF performed the experiment, analyzed the data, and wrote the manuscript. All authors contributed to the article and approved the submitted version.
Funding
The authors gratefully acknowledge the National Key R&D Research Program of China (Grant No. 2018YFB0905400), National Natural Science Foundation of China (Grant Nos. 51872277, 21606003, 51972067, 51802044, 51925207, and U1910210), the Fundamental Research Funds for the Central Universities (Grant No. WK2060140026), the DNL cooperation Fund, CAS (Grant No. DNL180310), and Guangdong Natural Science Funds for Distinguished Young Scholar (Grant No. 2019B151502039).
Conflict of Interest
The authors declare that the research was conducted in the absence of any commercial or financial relationships that could be construed as a potential conflict of interest.
Supplementary Material
The Supplementary Material for this article can be found online at: https://www.frontiersin.org/articles/10.3389/fenrg.2020.00200/full#supplementary-material
References
Bao, C., Guo, Y., Song, L., Lu, H., Yuan, B., and Hu, Y. (2011). Facile synthesis of Poly(vinyl alcohol)/α-titanium phosphate nanocomposite with markedly enhanced properties. Ind. Eng. Chem. Res. 50, 11109–11116. doi: 10.1021/ie200700t
Chen, N., Zhou, J., Kang, Q., Ji, H., Zhu, G., Zhang, Y., et al. (2017). Amorphous Vanadyl Phosphate/graphene composites for high performance supercapacitor electrode. J. Power Sources 344, 185–194. doi: 10.1016/j.jpowsour.2017.01.119
Chen, S., Wu, C., Shen, L., Zhu, C., Huang, Y., Xi, K., et al. (2017). Challenges and perspectives for NASICON-Type electrode materials for advanced sodium-ion batteries. Adv. Mater. 29:1700431. doi: 10.1002/adma.201700431
Deng, C., Xie, X., Han, J., Tang, Y., Gao, J., Liu, C., et al. (2020). A sieve-functional and uniform-porous kaolin layer toward stable zinc metal anode. Adv. Funct. Mater. 30:2000599. doi: 10.1002/adfm.202000599
Guo, C., Zhang, W., Liu, Y., He, J., Yang, S., Liu, M., et al. (2019). Constructing CoO/Co3S4 heterostructures embedded in n-doped carbon frameworks for high-performance sodium-ion batteries. Adv. Funct. Mater. 29:1901925. doi: 10.1002/adfm.201901925
Guo, X., Zhou, J., Bai, C., Li, X., Fang, G., and Liang, S. (2020). Zn/MnO2 battery chemistry with dissolution-deposition mechanism. Mater. Today Energy 16:100396. doi: 10.1016/j.mtener.2020.100396
Huang, H. L., Lii, K.-H., and Wang, S. L. (2014). Really understanding layered Vanadyl phosphate hydrates. J. Chin. Chem. Soc. 61, 199–206. doi: 10.1002/jccs.201300520
Hyoung, J., Heo, J. W., Chae, M. S., and Hong, S. (2019). Electrochemical exchange reaction mechanism and the role of additive water to stabilize the structure of VOPO4⋅2H2O as a Cathode material for potassium-ion batteries. Chemsuschem 12, 1069–1075. doi: 10.1002/cssc.201802527
Li, C., Wang, B., Chen, D., Gan, L., Feng, Y., Zhang, Y., et al. (2020). Topotactic transformation synthesis of 2D ultrathin GeS2 nanosheets toward high-rate and high-energy-density sodium-ion half/full batteries. ACS Nano 14, 531–540. doi: 10.1021/acsnano.9b06855
Li, Q., Chen, D., Tan, H., Zhang, X., Rui, X., and Yu, Y. (2020). 3D porous V2O5 architectures for high-rate lithium storage. J. Energy Chem. 40, 15–21. doi: 10.1016/j.jechem.2019.02.010
Liu, S., Zhu, H., Zhang, B., Li, G., Zhu, H., Ren, Y., et al. (2020). Tuning the kinetics of zinc-ion insertion/extraction in V2O5 by In situ polyaniline intercalation enables improved aqueous zinc-ion storage performance. Adv. Mater. 32:202001113. doi: 10.1002/adma.202001113
Peng, L., Zhu, Y., Peng, X., Fang, Z., Chu, W., Wang, Y., et al. (2017). Effective interlayer engineering of two-dimensional VOPO4 nanosheets via controlled organic intercalation for improving alkali ion storage. Nano Lett. 17, 6273–6279. doi: 10.1021/acs.nanolett.7b02958
Shi, H., Song, Y., Qin, Z., Li, C., and Sun, X. (2019). Inhibiting VOPO4⋅2H2O decomposition and dissolution in rechargeable aqueous zinc batteries to promote voltage and capacity stabilities. Angew. Chem. 58, 16057–16061. doi: 10.1002/anie.201908853
Tan, H., Xu, L., Geng, H., Rui, X., Li, C., and Huang, S. (2018). Nanostructured Li3V2(PO4)3 cathodes. Small 14:1800567. doi: 10.1002/smll.201800567
Tang, Y., Liu, C., Zhu, H., Xie, X., Gao, J., Deng, C., et al. (2020). Ion-confinement effect enabled by gel electrolyte for highly reversible dendrite-free zinc metal anode. Energy Storage Mater. 27, 109–116. doi: 10.1016/j.ensm.2020.01.023
Verma, V., Kumar, S., Manalastas, W. Jr., Zhao, J., and Chila, R. (2019). Layered VOPO4 as a cathode material for rechargeable zinc-ion battery: effect of polypyrrole intercalation in the host and water concentration in the electrolyte. ACS Appl. Energy Mater. 2, 8667–8674. doi: 10.1021/acsaem.9b01632
Wang, B., Ang, E. H., Yang, Y., Zhang, Y., Geng, H., Ye, M., et al. (2020). Interlayer engineering of molybdenum trioxide toward high-capacity and stable sodium ion half/full batteries. Adv. Funct. Mater. 30:2001708. doi: 10.1002/adfm.202001708
Wang, J., Tan, S., Xiong, F., Yu, R., Wu, P., Cui, L., et al. (2020). VOPO4⋅2H2O as a new cathode material for rechargeable ca-ion batteries. Chem. Commun. 56, 3805–3808. doi: 10.1039/d0cc00772b
Wang, Q., Xu, J., Zhang, W., Mao, M., Wei, Z., Wang, L., et al. (2018). Research progress on vanadium-based cathode materials for sodium ion batteries. J. Mater. Chem. A 6, 8815–8838. doi: 10.1039/C8TA01627E
Wang, Q., Zhang, W., Guo, C., Liu, Y., Wang, C., and Guo, Z. (2017). In situ construction of 3D interconnected FeS@Fe3C@ graphitic carbon networks for high-performance sodium-ion batteries. Adv. Funct. Mater. 27:17003390. doi: 10.1002/adfm.201703390
Xie, X., Liang, S., Gao, J., Guo, S., Guo, J., Wang, C., et al. (2020). Manipulating the Ion-transfer kinetics and interface stability for high-performance zinc metal anodes. Energy. Environ. Sci. 13, 503–510. doi: 10.1039/c9ee03545a
Xiong, P., Zhang, F., Zhang, X., Wang, S., Liu, H., Sun, B., et al. (2020). Strain engineering of two-dimensional multilayered heterostructures for beyond-lithium-based rechargeable batteries. Nat. Commun. 11, 3297–3297. doi: 10.1038/s41467-020-17014-w
Yang, D., Liu, C., Rui, X., and Yan, Q. (2019). Embracing high performance potassium-ion batteries with phosphorus-based electrodes: a review. Nanoscale 11, 15402–15417. doi: 10.1039/C9NR05588F
Yang, H., Xu, G., Wei, X., Cao, J., Yang, L., and Chu, P. K. (2018). Ultrafast hetero-assembly of monolithic interwoven V2O5 nanobelts/carbon nanotubes architectures for high-energy alkali-ion batteries. J. Power Sources 395, 295–304. doi: 10.1016/j.jpowsour.2018.05.081
Yang, Y., Zhu, H., Xiao, J., Geng, H., Zhang, Y., Zhao, J., et al. (2020). Achieving ultrahigh-rate and high-safety Li+ storage based on interconnected tunnel structure in micro-size niobium tungsten oxides. Adv. Mater. 32:1905295. doi: 10.1002/adma.201905295
Yu, Y., Zhang, X., Rui, X., Chen, D., Tan, H. T., Yang, D., et al. (2019). Na3V2(PO4)3: an advanced cathode for sodium-ion batteries. Nanoscale 11, 2556–2576. doi: 10.1039/C8NR09391A
Zhu, Y., Peng, L., Chen, D., and Yu, G. (2016). Intercalation pseudocapacitance in ultrathin VOPO4 nanosheets: toward high-rate alkali-ion-based electrochemical energy storage. Nano Lett. 16, 742–747. doi: 10.1021/acs.nanolett.5b04610
Zhao, L., Zhao, H., Du, Z., Wang, J., Long, X., Li, Z., et al. (2019). Delicate lattice modulation enables superior na storage performance of Na3V2(PO4)3 as both an anode and cathode material for sodium-ion batteries: understanding the role of calcium substitution for vanadium. J. Mater. Chem. A 7, 9807–9814. doi: 10.1039/C9TA00869A
Zhou, K., Zhang, Q., Wang, B., Liu, J., Wen, P., Gui, Z., et al. (2014). The integrated utilization of typical clays in removal of organic dyes and polymer nanocomposites. J. Clean. Prod. 81, 281–289. doi: 10.1016/j.jclepro.2014.06.038
Zhou, K., Zhou, G., and Yuan, H. (2016). Ultrathin 2D VOPO4 nanosheets: a novel reinforcing agent in polymeric composites. RSC Adv. 6, 100344–100351. doi: 10.1039/C6RA24111E
Keywords: sodium ion battery, cathode material, VOPO4⋅2H2O, nanosheets, high performance
Citation: Zhang X, Yang D, Liu W, Feng Y, Rui X and Yu Y (2020) VOPO4⋅2H2O Nanosheet Cathode for Enhanced Sodium Storage. Front. Energy Res. 8:200. doi: 10.3389/fenrg.2020.00200
Received: 19 June 2020; Accepted: 29 July 2020;
Published: 19 August 2020.
Edited by:
Jianfeng Mao, University of Wollongong, AustraliaReviewed by:
Xuezhang Xiao, Zhejiang University, ChinaChaofeng Zhang, Anhui University, China
Qinghong Wang, Jiangsu Normal University, China
Copyright © 2020 Zhang, Yang, Liu, Feng, Rui and Yu. This is an open-access article distributed under the terms of the Creative Commons Attribution License (CC BY). The use, distribution or reproduction in other forums is permitted, provided the original author(s) and the copyright owner(s) are credited and that the original publication in this journal is cited, in accordance with accepted academic practice. No use, distribution or reproduction is permitted which does not comply with these terms.
*Correspondence: Xianhong Rui, xhrui@gdut.edu.cn; Yan Yu, yanyumse@ustc.edu.cn